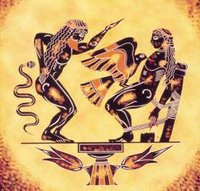
Despite its large metabolic load, the liver is a largely quiescent organ in terms of cell proliferation, with only 0.01-0.001% of hepatocytes undergoing mitosis at any given time. This cellular turnover can be altered by toxic liver injury or surgical resection, which results in sudden, massive hepatocyte proliferation, producing recovery of functional liver mass within two weeks after the loss of up to two-thirds of the liver.
Experimental models of liver regeneration
Liver resection or partial hepatectomy (PH) reduces liver mass, but leaves few injured cells. Although the remaining hepatic segments are subjected to increased portal blood flow and pressure following PH, PH is still the closest approximation of pure liver regeneration unaccompanied by cellular injury and is therefore the preferred in vivo model to study.
Immediately following PH or other liver injury, hepatocytes exit the resting state of the cell cycle (G0), enter G1, traverse to DNA synthesis or S phase, and ultimately undergo mitosis, with a first peak of DNA synthesis occurring in the parenchymal cells (hepatocytes and bile duct epithelial cells). Non-parenchymal cells display a first peak of proliferation approximately 12-24 hours after the parenchymal cells. The degree of hepatocyte proliferation is directly proportional to the degree of injury, this being that any injury greater than about 10% will result in proliferation of cells throughout the liver.
Total liver mass is tightly modulated by pro-mitotic and apoptotic mechanisms, and possesses the remarkable ability to grow or shrink quickly in order to meet metabolic demands following hepatocellular injury or in response to situations of excess functional mass. An example of this is small-for-size liver transplants, where the liver will rapidly grow to accommodate the needs of the larger host, while larger livers transplanted into smaller hosts will undergo apoptosis to reach the normal liver to body mass ratio of 2.5%.
Although liver function appears virtually normal following PH, there can be no survival after liver injury without regeneration. In all animals studied, including humans, the failure to restore functional hepatic mass invariably leads to death. Impairment of liver regeneration is observed in some disease states (including non-alcoholic fatty liver disease, cirrhosis, chronic hepatic infection, malnutrition) with increased cell death, delayed mitosis and slower return of normal hepatic mass.
Intrinsic regenerative capacity of the liver
Serial transplant studies in mice suggest that the hepatocyte possesses an essentially limitless regenerative capacity, as shown by the works of Brinster and colleagues, and by Grompe.
In contrast to the impressive proliferative potential of the hepatocyte, the regenerative potential of the liver is limited. Clinical observations have determined that the upper-limit for single liver resection is approximately 70-80% in humans although if the functional liver mass returns to pre-resective levels, repeat resection of as much as 50% of the regenerated liver can be tolerated.
Evidence for the role of circulating factors in liver regeneration

Starzl and coworkers identified essential roles for several humoral factors, including islet cell-derived insulin along with an essential role for other gut-derived agents in dogs. This has resulted in the identification of factors involved in: 1) initiating or “triggering” the hepatic response to injury; 2) inducing hepatocyte proliferation; and 3) terminating cell proliferation once normal liver mass is restored have been identified.
Priming is accomplished by the release of preformed cytokines that subsequently activated transcription factor complexes and allow the cell to exit G0 to G1 of the cell cycle. After the priming event, increased expression of pro-mitogenic growth factors and their receptors is observed concomitant with down-regulation of hepatostatic factors and receptors. Facilitated by co-mitogens, growth factor signaling permits progression of the primed hepatocyte through the cell cycle.
Cessation of liver growth once normal mass is reached is also regulated by multiple pathways including increased expression and responsiveness to hepatostatic factors, or tonic growth inhibitors.
The secreted and soluble factors involved in the response to PH can be classified using the following five categories:
1) Priming Factors
2) Growth Factors
3) Co-mitogens
4) Tonic Growth Inhibitors
5) Suppressors of Growth Inhibitors
Tumor Necrosis Factor (TNF): TNF, a cytokine with pleiotrophic effects is required for normal liver regeneration. , TNF can induce DNA synthesis in cultured hepatocytes., as a priming agent which renders hepatocytes responsive to growth factors.
Interleukin-6 (IL-6): is a multifunctional proinflammatory cytokine involved in a variety of host defenses and pathological processes. IL-6 acts on the cell by binding the IL-6 receptor alpha chain (IL-6Ra, also known as gp80), inducing dimerization of the gp130 receptor and subsequent intracellular signaling partners. IL-6 is expressed both in the liver and the gut following liver resection.
Hepatocyte Growth Factor (HGF): HGF was isolated based upon its ability to induce DNA synthesis in cultured hepatocytes, but is now known to have pleiotrophic effects on many other tissues. Shown to weakly induce hepatocyte proliferation. Two reports of HGF transgenic mice, have led Fausto and others to postulate that normal hepatocytes in vivo have a low sensitivity to growth factors, and can only respond to such factors after gaining replicative competence by priming, or activation of the transcription factor complexes NF-kB, AP-1, and STAT3 followed by expression of immediate early genes.
Epidermal Growth Factor (EGF) and Transforming Growth Factor-alpha (TGF-): EGF stimulates the growth of various epidermal and epithelial tissues in vivo and in vitro, and of some fibroblasts in cell culture.
Insulin and Glucagon: Insulin is regarded as an anabolic, growth-promoting hormone, while glucagon has opposing activities, exerting a catabolic effect. Insulin and glucagon, particularly when administered together, have been shown to accelerate liver regeneration after PH.
Activin and Follistatin: Activin, another member of the TGF-ß superfamily, has potent growth inhibitory effects on hepatocytes
Chemokines: a diverse group of approximately 30 known members involved in a wide variety of inflammatory processes. Chemokines have been shown to facilitate recovery following toxic liver injury, and may play a role in regulating hepatocyte proliferation either directly, or indirectly through induction of HGF expression.
Prostaglandins: PGE2, prostacyclin, and thromboxane, may be involved in growth regulation after PH. Prostaglandins are synthesized from arachidonic acid released from membrane phospholipid by phospholipase A2. Arachidonic acid is oxidized by cycloxygenases (COX) to generate the precursor PGH2, which is further metabolized to form prostaglandins, thromboxanes, and prostacyclins. Although COX inhibitors have not yet been demonstrated to block regeneration in humans, these data suggest that care should be exercised in the use of non-steroidal anti-inflammatory agents, such as indomethacin, following major liver resection.
Steroids and Thyroid Hormone: steroids have been noted to regulate both cell growth as well as fatty content of hepatocytes. Sex steroids are known to induce transient hepatocellular proliferation and improve fatty acid metabolism. Pre-treatment of rats with 17ß-estradiol induces hepatocyte DNA synthesis in vitro and accelerates liver regeneration in vivo. Thyroid hormone (T3) is also a powerful inducer of hepatocyte proliferation in vitro and in vivo.
Glucocorticoids inhibit hepatocyte proliferation as well as decrease the expression of a number of the genes involved in fatty acid metabolism. Thus the use of glucocorticoids may impair liver regeneration both by aggravating steatosis and inhibiting growth.
Intracellular signals involved in liver regeneration
Identified over 200 genes associated with signaling processes in addition to the many hundreds of genes also being expressed and modulating the regenerative response.
Priming Pathways:
TNF Receptor Signaling: TNF renders the hepatocyte competent to respond to growth stimuli.
Nitric Oxide: highly reactive molecule synthesized from arginine by nitric oxide synthase (NOS). Three distinct isoforms of NOS exist, constitutive NOS (cNOS) (which includes brain (bcNOS) and endothelial NOS (ecNOS)) and inducible NOS (iNOS). In the case of compensatory hepatic growth both the cNOS and iNOS isoforms are thought to play a critical role in successful hepatic regeneration following PH.
It appears that iNOS induction occurs predominantly in the hepatocytes and coincides with the pre-cell replication phase (6-8 hours). The induction of iNOS is cytokine dependent and has been demonstrated to coincide with the induction of IL-6 inducible transcription factors. NO plays an essential role on hepatocyte repopulation of the liver.
Growth Promoting Pathways: Sub-divided into four groups: guanine nucleotide protein (G-protein) coupled-receptors, receptors with intrinsic tyrosine kinase activity, receptors that recruit cytoplasmic kinases, and nuclear hormone receptors. When activated subtypes result in expression of growth related genes. essential for cell proliferation.
Growth-Inhibitory Pathways: Cessation of liver growth after regeneration and indeed perhaps the tonic growth-inhibition of the liver in the normal state is at least partly mediated by the TGF-ßs and the Activins, all members of the TGF-ß superfamily. Recent evidence suggests that TGF-ß/Activin signaling in early liver regeneration might be blocked by increased expression of the Smad pathway inhibitors SnoN and Ski.
Interactions of signaling pathways during liver regeneration
The presence of numerous diverse yet specific ligands and receptors in the initiation, propagation and termination of the process of hepatic regeneration is an essential factor in the successful and complete restoration of hepatic mass. But, hepatic regeneration cannot proceed unless the activated receptors each communicate successfully with the cell nucleus to allow the regulation of transcriptional factors and subsequent de novo DNA synthesis, cell proliferation and differentiation. Receptor activation and regulation of intracellular pathways represent common means by which distinct receptors can communicate with the nucleus, and are laid out as linear signal transduction pathways.
Apoptosis during liver regeneration
Initiation of proliferation may be difficult or impossible for certain diseased hepatocytes. Cell death under these circumstances will occur by a process of preprogrammed cell death, termed apoptosis. In vivo apoptosis may be activated by engagement of the socalled "death receptors" by their ligands, including Fas ligand, which is a protein secreted by lymphocytes, TNF, and TNF-related molecules or by activation of serine-threonine kinase receptors by members of the TGF-ß superfamily, including Activin and TGF-b.
Alternatively, apoptosis may be activated secondarily to mitochondrial release of cytochrome C after drug-induced injury. During activation, a number of caspase enzymes and intracellular signals of the bcl2 family (Bad, Bak, Bax) become activated. In turn, these lead to digestion of the nucleus and destruction of the cell’s DNA, primarily through the direct action of caspase 3. During successful liver regeneration, the expression and activity of pro-apoptotic pathways is inhibited through increased expression of anti-apoptotic Bcl-2 family members (notably Bcl-2 and Bcl-XL).
Numerous factors have been implicated in activating anti-apoptotic signals in the liver inducible nitric oxide synthase and IL-6. Certain disease states lead to an impaired ability to down regulate apoptotic pathways in the liver, like steatosis, for example. Ischemic preconditioning, i.e. providing a short period of ischemia followed by reperfusion of the liver prior to undergoing liver resection, has been shown both in animal and human studies to decrease apoptosis associated with liver resection both in hepatocytes as well as in hepatic endothelial cells.
Ischemic preconditioning has been demonstrated to decrease post-resective serum markers of liver injury in both animal and human studies. This phenomenon is even more pronounced if steatotic liver is treated. Clavien and coworkers demonstrated success in humans undergoing liver resection with an ischemic preconditioning protocol involving 10 minutes of ischemia followed by 10 minutes of reperfusion prior to hepatic resection. The pathways that elicit this protective response are still under active investigation, although work by Diehl and colleagues and Jaeschke et al. implicates nitric oxide, TNF, and neutrophils in the oxidant and protease-mediation of hepatocellular injury after experimental ischemia/reperfusion injury.
Effects of steatosis, steatohepatitis, fibrosis and cirrhosis on liver regeneration

Steatohepatitis is therefore a relative contraindication to major hepatic resection or use for transplantation. Mortality following resection in these livers generally occurs within days after surgery when regeneration fails and liver failure and hepatocyte apoptosis occurs. Whole organ liver abnormalities that result from steatosis include reduction of the sinusoidal space, which in turn may result in abnormal blood flow. Lipid accumulation is also associated with hepatocyte mitochondrial damage. This is due to free radical injury from abnormal fatty acid oxidation and accumulation of dicarboxylic fatty acids during intermediary metabolism. Abnormalities in cytochrome p450 (CYP) induction have also been implicated in fatty liver and steatohepatitis.
Hepatic fibrosis, biochemically, represents the partially reversible accumulation of a fibrillar collagen matrix and relative loss of the normal type IV collagen matrix basement membrane. This phenomenon has been found to begin in the subendothelial space and is due largely to the secretion of abnormal collagen by stellate cells. Abnormal regeneration is manifest in the fibrotic liver due to the chronically impaired diffusion of nutrient and hepatotrophic factors through the abnormal fibrillar basement membrane. Impaired hepatocyte function results from absence of normal trophic factors. Furthermore, the scar contracture of cirrhosis also becomes a physical barrier to compensatory hyperplasia following resection.
Effects of commonly prescribed drugs on liver regeneration
Effects of various medications on liver regeneration have become recognized. Of particular concern are herbal supplements, which contain numerous, often poorly-defined biochemicals including numerous agents that may stimulate nuclear receptors to induce steatosis or even NASH. Cessation in patients about to undergo liver resection is suggested.
Beta-blockers inhibit the effects of epinephrine, an important comitogen for hepatocytes. They also decrease portal flow and, as such, have a critical role in the management of portal hypertension. The cellular mechanism involves decreased G-protein coupled signaling with impaired adenylate cyclase, cAMP and down-stream signals. reduction of portal blood flow may restrict the perfusion of enterically-derived factors such as TNF and EGF into the liver.
J Gastroenterol Hepatol 1996; 11:466–470; Arch Surg 2000;135:1353–1358; Semin Liver Dis 2002;22:137–144; Radiology 1999;210:467–477; J Surg Res 2001;96:289–295; Liver Transplant Surg 1996;2:233–234; Hepatology 1991;13:1–5; J Hepatol 1996;24:385–390; Hepatology 1994;19:489–497; Hepatology 2003;38:326–334; Ann Surg 2002;235:863–871.
0 Comments:
Post a Comment